The conversion efficiency of solar cell depends on the material absorption and charge transport properties. When using only one pn-junction material the theoretical conversion efficiency, so called Shockley–Queisser limit, cannot exceed 30%. For such single junction solar cell, silicon (Si) and gallium arsenide (GaAs) are nearly ideal materials exhibiting peak efficiencies of 26.7% and 29.1%, respectively [2, 3].
In a single junction solar cell, the conversion efficiency is limited because part of the photon energy is converted into heat (photons with energy much larger than the bandgap) and transmission losses (photon with energy lower than the bandgap). The ratio of thermalization to transmission losses depends on the absorption edge of solar cell material set by the band gap. If band gap energy is high (~2 eV), the thermalization losses are low, but the transmission losses are high. On the other hand, for narrow band gap materials (~0.7 eV), the thermalization losses are high but the transmission losses are low. Indeed if semiconductors of different bandgaps can be combined in the same device, then one would gain an ability to reduce the two major loss mechanisms and hence improve the conversion efficiency. This combination principle is the foundation for the operation of a multi-junction solar cell (see figure), which theoretically could reach a conversion efficiency as high as 86% for an infinite number of junctions [4]. In terms of practical demonstration, there are other limitations, for example imposed by the availability of materials that can be stacked without defects, that makes the real-world efficiencies still to remain well below 50%.
Multijunction solar cells are typically fabricated by metal organic chemical vapour deposition (MOCVD) or by molecular beam epitaxy (MBE) and are inherently more expensive than the widely spread solar cells. Hence, they find use in specialized applications, such as space satellites, space stations, and terrestrial concentrated photovoltaic (CPV) power plants. For space, the III-V cells are used due to their excellent radiation hardness and high efficiency. For terrestrial CPV power plants, the key driving force of using III-V multijunction solar cells has been the possibility to use high concentrations (~1000 suns) enabling an additional mechanism to increase the superior efficiencies.
The goal of AMETIST project
In AMETIST project, we aim at better utilization of the solar spectrum, enabling efficiencies approaching practical limits by engineering advanced III-V optoelectronic materials and heterostructures. The work is strongly motivated by the global need for renewable energy sources. To this end, AMETIST framework is based on three vectors of excellence in:
i) material science and epitaxial processes,
ii) advanced solar cells employing nanophotonic concepts, and
iii) new device fabrication technologies.
Novel materials (e.g. GaInNAsSb), providing absorption in the broad spectral range from 0.7 eV to 1.4 eV, will be synthesized and monolithically integrated in tandem cells with more than to 4 junctions. Nanophotonic structures for light-trapping, involving spectral and spatial control of solar radiation, will be developed to further enhance the absorption. To ensure a high long-term impact, the project will validate the use of state-of-the-art MBE processes for fabrication of economically viable ultrahigh-efficiency solar cells. The ultimate efficiency target is ~55%. The work will also bring new developments for more efficient space photovoltaic systems.
References
[2] https://www.nrel.gov/pv/cell-efficiency.html
[3] M. A. Green et al., Solar cell efficiency tables (Version 53) (https://doi.org/10.1002/pip.3102)
[4] A De Vos, Detailed balance limit of the efficiency of tandem solar cells, Journal of Physics D: Applied Physics, Volume 13, Number 5
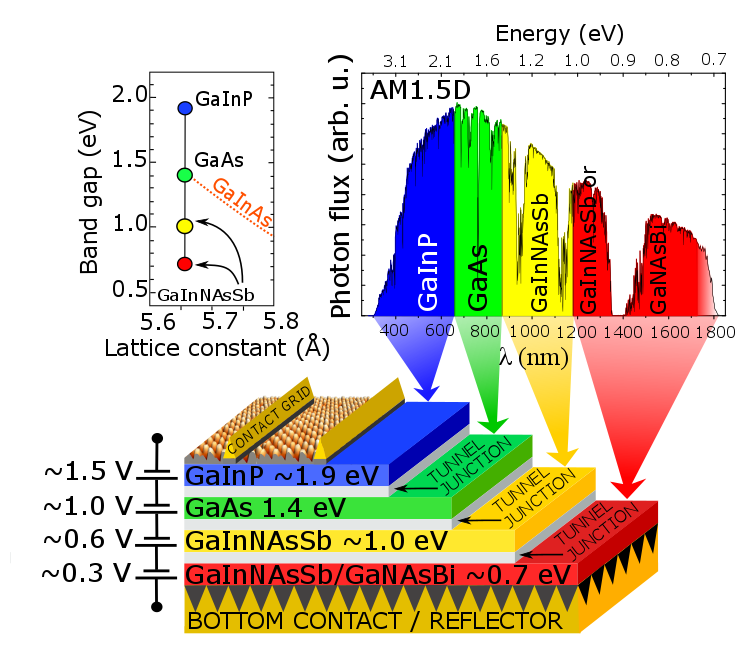
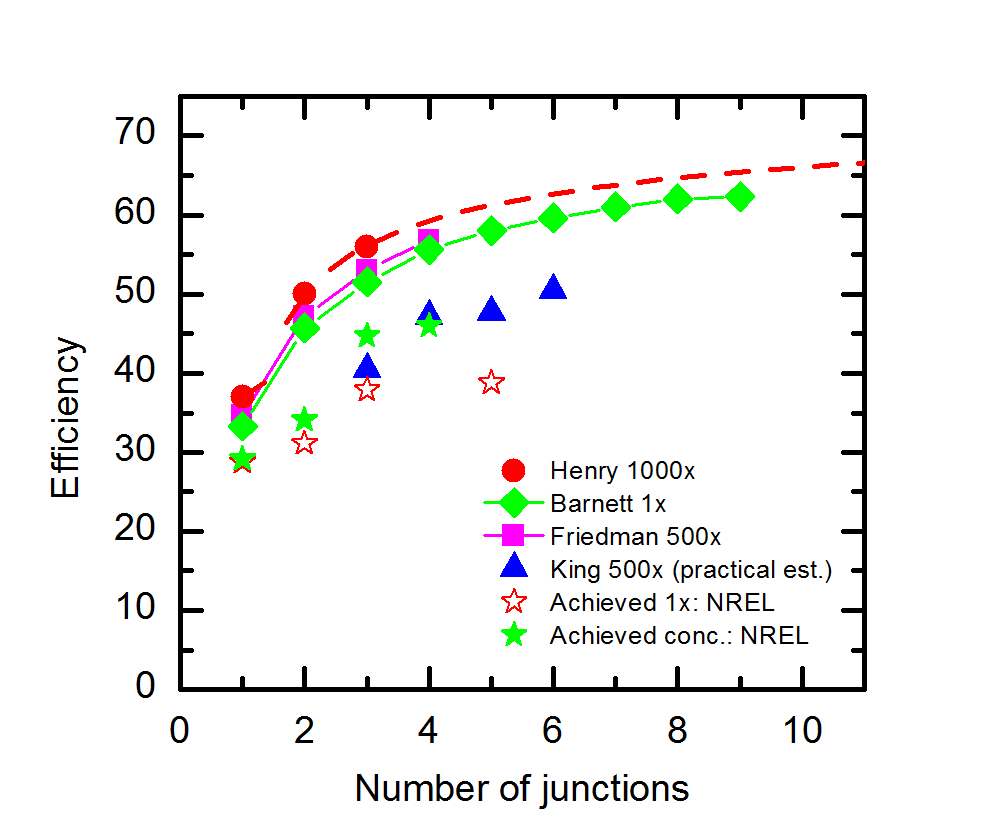
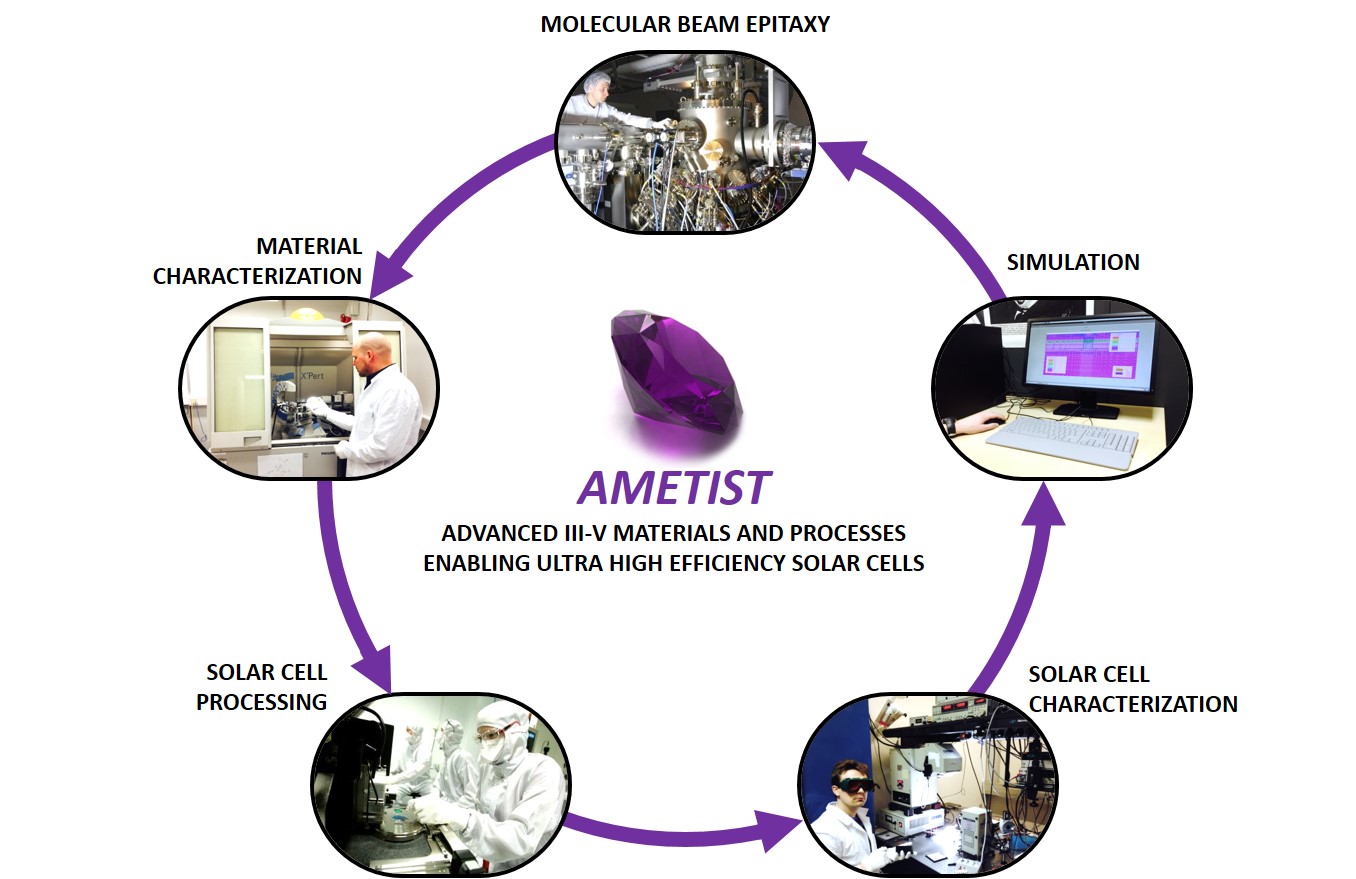